COVID-19 vaccines
Fast vaccine stats
As of December 27th, 2022:
- People fully vaccinated globally: 5,057,005,921 people
- People fully vaccinated in the United States: 229,135,170 people
- Allergic responses to vaccination in the US: ~4.5 cases per million doses
- Deaths from COVID-19 mRNA vaccination (Pfizer & Moderna) in the US: 0* people
- Deaths from COVID-19 AstraZeneca vaccination in the UK: 19** people (this early data was out of 20 million doses = a low risk of ~1/1,000,000)
- Deaths from COVID-19 Johnson & Johnson vaccination: 3** people (this early data was out of 7 million doses = a low risk of ~1/2,300,000)
- Deaths from COVID-19 globally: 6,681,843 people
- Deaths from COVID-19 in the US: 1,090,833 people
"Deaths from COVID" include when other complications resulted in death during a COVID infection.
*113 deaths were reported in the first month of vaccination, but the deaths were not caused by the vaccine itself.
**Cause of death is not confirmed, but suspected to be related to the vaccine. Additional cases of blood clotting are under investigation but have not caused additional deaths. All medical treatments carry some risk, even if it's small. Always contact your medical professional if you have questions about a treatment.
Data from ourworldindata.org and CDC.gov
Variants of the virus
As of January 1st, 2023, the most well-known variants of the virus SARS-CoV-2 include:
- B.1.1.529 - BA.5, XBB 1.5 (Omicron & Omicron subvariants): Believed to have evolved in either Europe or Africa, South African scientists were the first to alert the rest of the world to Omicron's existence; found in well over 100 countries and quickly spreading; it is the dominant variant in the United States. It spreads more easily than other variants, and causes less severe infection generally, though severe infections can still occur; breakthrough infections are common, but for people who are vaccinated, most infections are mild. New subvariants like XBB 1.5 are emerging that we are still learning about. New Pfizer and Moderna vaccines and boosters help prevent severe disease against Omicron, so getting vaccinated is still very important. This variant can still be spread by the vaccinated and even the recently boosted, so masks are also encouraged for everyone, especially during times of high transmission.
- BQ.1 - BQ.1.1 (Omicron subvariants): New subvariants of Omicron BA.5, these variants have many new mutations and the numbers are rising in many countries. The mutations suggest it will be less responsive to the original COVID-19 vaccines, but the new Omicron-specific vaccine booster should offer some protection from severe disease. Boosting is especially important with these variants, as many currently available antibody treatments will likely not be useful. Masks are strongly encouraged for everyone to avoid these variants, especially during times of high transmission.
- B.1.617 (Delta/"double-mutant" variant): No longer a variant of concern. First found in India, found in over 170 countries; researchers have found that it spreads more easily and may be more dangerous than the original virus; the available vaccines are very helpful against this variant, though breakthrough cases seem to be higher (whether this is due to time since vaccination or the delta variant is unknown).
- B.1.621 (Mu): No longer a variant of concern. First found in Columbia, found in over 35 countries; this variant did not spread as well as the Delta variant.
- B.1.1.7 (Alpha): No longer a variant of concern. First found in the UK; found in over 100 countries. This variant was thought to spread up to 50% more easily than the original virus and to cause more severe disease; vaccines work well against this variant.
- B.1.351 (Beta): No longer a variant of concern. First found in South Africa; found in over 80 countries; various mutations change the shape of the spike protein; these mutations seem to reduce the ability of current vaccine-based antibodies to fight the virus by about 30%; all available vaccines can still reduce severe disease from this variant; mRNA vaccines can reduce mild to moderate disease from this variant.
- P.1 (Gamma): No longer a variant of concern. First found in Brazil; found in at least 37 countries; shares at least one mutation with Beta that slightly changes the spike protein; appears to spread more easily than most other variants.
Some companies producing vaccines are already working on altered versions of the approved vaccines, to help fight specific variants if necessary.
In 2020, the race to make a vaccine against SARS-CoV-2, the virus that causes COVID-19, was a top priority for scientists. With so many types of vaccines, it's sometimes hard to understand how each one works. Let’s take a look at two main types: mRNA and viral vector vaccines.
The news was reporting these as new types of vaccines. But, it is important to know that both types of vaccines have been researched for decades to protect against many types of viruses, like Zika and Ebola. Current mRNA vaccines and viral vector vaccines that help protect against COVID-19 both contain genetic material that carries instructions to our cells. These instructions teach our cells to make a protein. The protein trains the immune system to fight against the virus that causes COVID-19. However; both types of vaccines differ in their ingredients, the type of genetic material used, and the way they deliver them.
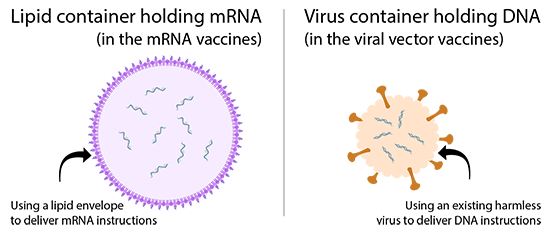
mRNA vaccines
Some of the first vaccines approved for public use against COVID-19 were mRNA vaccines. The mRNA vaccines from Pfizer-BioNtech and Moderna both give close to 95% defense against symptoms from SARS-CoV-2. Both vaccines need two doses at least 21 days apart (Pfizer-BioNtech) or 28 days apart (Moderna). In healthy recipients, both vaccines give close to 100% protection from severe COVID-19.
When thinking about “effectiveness” of the vaccine against COVID symptoms versus severe disease, what exactly does that mean? Effectiveness of the vaccines against COVID-19 "symptoms" refers to any symptoms experienced, even if they were very mild. Effectiveness against "severe COVID-19" or “severe disease” refers to more serious symptoms that could require medical attention. The most important thing to understand about all the approved vaccines is that they ALL offer ~100% protection from death caused by COVID-19, even if someone experiences severe symptoms. This highlights the importance of getting vaccinated, and not waiting for one particular type of vaccine, whether you get an mRNA vaccine, or a viral vector vaccine. Whichever you might get, let's take a closer look at how they work.
How mRNA vaccines work
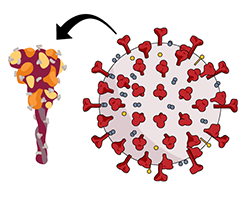
mRNA, or messenger ribonucleic acid, is normally in your cells and gives cells instructions to make proteins that cells need to function. COVID-19 mRNA vaccines contain mRNA that gives your cells instructions to make a protein called the "spike" protein of SARS-CoV-2. The spike protein is on the surface of SARS-CoV-2 and is the part of the virus that attaches to a cell’s surface to enter.
The spike protein on its own is harmless, and it cannot give you the virus. COVID-19 mRNA vaccines only make the spike protein of the virus and do not carry any live virus, which means someone cannot get COVID-19 from the vaccine. The mRNA itself is inside small capsules made of fats called “lipid nanoparticles.” These are taken up by your immune cells after you are vaccinated.
Once in the immune cell, the lipid capsules release the mRNA into the cytoplasm of the cell. The cell reads the mRNA's instructions and makes the SARS-CoV-2 spike protein. Next, your cell displays parts of this spike protein on its surface. This way, other cells are aware of the protein, and your immune system can respond to it. The mRNA never enters your cell's nucleus, the area where DNA is stored. All of the mRNA and other ingredients of these vaccines only stay in the body temporarily and do not alter your DNA.
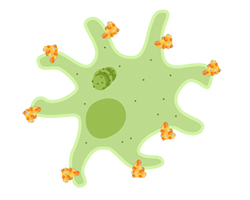
During an immune response to the spike protein, your body makes antibodies that attach to the protein. The immune cells that make those antibodies are replicated to make more until enough antibodies can be made to fight the virus. Other immune cells are recruited to find and kill the proteins marked with antibodies. The fight against the spike proteins in the vaccine is a way that your body learns how to beat the spike.
As a part of this process, your body will also make memory immune cells from the cells that were able to fight the protein. These cells should be able to recognize the spike proteins in the future, and make antibodies to the full virus, or kill infected cells, if the virus ever gets into the body. This process is much faster and more successful when your immune system has learned about the spike protein from a vaccine.
Long-term learning with vaccines
If SARS-CoV-2 enters the body after someone is fully vaccinated, antibodies made to fight the spike protein will quickly attach to the virus and act like little red flags. They will alert other immune cells to clear the virus. When attached to the virus, they can also physically block it from entering and infecting cells. This provides protection from infection for those vaccinated against SARS-CoV-2. Because your body recognizes important parts of the virus, it can fight it off much faster than it would have before, giving the virus little chance to cause a bad infection.
Viral vector vaccines
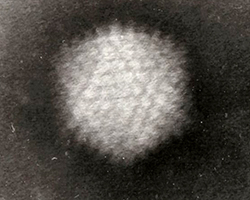
Some other vaccines approved for use against SARS-CoV-2 are viral vector vaccines. Viral vectors are versions of a harmless virus that are used to deliver genetic material from another virus to our cells. You can think of it as wrapping a tiny bit of harmless genes from one virus we want to protect against in the covering of another virus that won’t hurt us. The genetic material from the virus we want to protect against (in this case, SARS-CoV-2) holds instructions to make specific proteins in a cell.
Oxford-AstraZeneca and Johnson & Johnson vaccines against COVID-19 use viral vectors. Oxford-AstraZeneca’s vaccine caused at least a 63% reduction in symptoms from SARS-CoV-2 and close to 100% protection against severe SARS-CoV-2 after two doses. Johnson & Johnson’s vaccine is a single shot and is at least 66% effective overall in preventing moderate to severe COVID-19. The Johnson & Johnson vaccine is at least 85% effective at preventing severe COVID-19 and 100% effective at preventing death from COVID-19.
How do viral vector vaccines work?
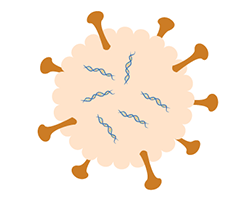
In the case of COVID-19 viral vector vaccines, the genetic material also contains instructions that tell some of your immune cells to make the spike protein. However; its instructions are made up of DNA instead of RNA. Upon vaccination, the viral vector will enter a cell, as a normal virus would. The vector will bring the DNA that contains instructions to produce the spike protein into the nucleus of the cell. There, the cell will begin to make mRNA that moves to the outside of the nucleus and into the cytoplasm. From there the spike protein will be made based off of the mRNA that was produced. The cell then displays parts of the spike protein on its surface, where the immune system can recognize it as an invader and launch a response.
Like mRNA vaccines, viral vector vaccines do not contain live SARS-CoV-2. So, someone cannot get COVID-19 from getting the vaccine. Additionally, the DNA contained in the viral vectors has been modified. The vector can enter cells but the genes needed for the virus to replicate have been deleted from the DNA. This means the viral vectors are not able to replicate in a cell, so they cannot cause disease. The DNA in the vector does not affect our cells because it is cleared after the protein is produced. These vaccines are more stable than mRNA and for this reason, may be kept for longer periods at higher temperatures than mRNA vaccines. This means these vaccines could be distributed to poorer countries without the ability to freeze the vaccine for long periods of time.
What to expect after getting vaccinated
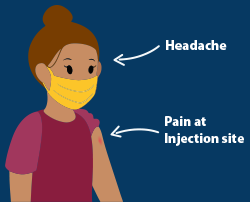
Vaccines go through careful safety testing before they are approved for public use in the United States. For COVID-19 vaccines, long-term safety data will continue to be collected. All of these vaccines may cause some cold-like symptoms. The most common side effects of the COVID-19 vaccines are pain at the site of injection, and possible headache, slight fever, and feeling tired.
It is important to know these symptoms are due to your immune system reacting to the vaccine and preparing to fight off the actual virus if the body ever comes in contact with it. For this reason, people should get the vaccine when they are eligible, to reduce the spread of COVID-19. Make sure not to take any unnecessary medications before or right after you get your vaccine. Some medications, like certain pain killers, may reduce the effectiveness of your immune system to launch a full attack during this training period.
Vaccines and variants
It is natural for viruses to mutate if they have spread from one person to another for some time. Since the Summer of 2020, we have seen this happening in SARS-CoV-2. Many people are worried that current COVID-19 vaccines may not protect against newly detected SARS-CoV-2 variants. So what does the science say about the variants?
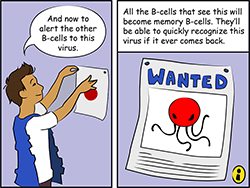
Most variants have mutations in the virus’s spike protein. It is possible that some of these mutations may make the virus more contagious than the initial pandemic strain. This means that if a person is infected with a new variant, they could infect more people when sick than if they had the original pandemic strain. It is important to note that some SARS-CoV-2 mutations can affect vaccine effectiveness. However, these mutations are in only part of the spike protein, they don’t affect the entire spike.
Current COVID-19 vaccines train the body to fight the entire spike protein. If someone is vaccinated and comes into contact with a variant, they should still have antibodies that work on the spike protein parts that were not mutated. So those antibodies should still help fight the mutated virus, even though in some cases they won’t be as effective. This does not mean people will not get sick at all from variants, but they will have better outcomes than had they not been vaccinated. These variants also provide a good reminder for many people that it is still important that we socially distance, use masks, and wash our hands, even after we've been vaccinated.
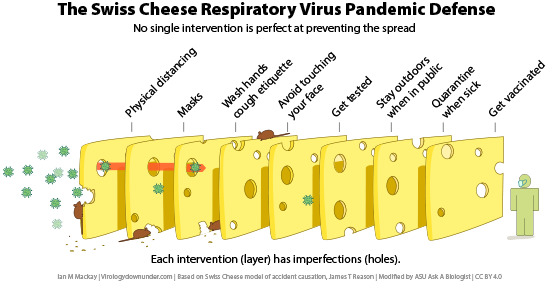
Vaccination with any of the available COVID-19 vaccines should still give some protection from the variants that have been discovered to date. For this reason, even if the vaccines are less effective against variants, some protection is better than no protection. It is important to get the COVID-19 vaccine to help stop the spread of all COVID-19 variants.
References
https://www.jnj.com/johnson-johnson-covid-19-vaccine-authorized-by-u-s-f...
https://www.astrazeneca.com/media-centre/press-releases/2021/covid-19-va...
https://www.cdc.gov/coronavirus/2019-ncov/more/science-and-research/scie...
https://www.who.int/en/activities/tracking-SARS-CoV-2-variants/
Read more about: Vaccine science
Bibliographic details:
- Article: COVID-19 vaccines
- Author(s): Michelle Di Palma
- Publisher: Arizona State University School of Life Sciences Ask A Biologist
- Site name: ASU - Ask A Biologist
- Date published: 25 Feb, 2021
- Date accessed: 15 June, 2025
- Link: https://askabiologist.asu.edu/covid-vaccine
APA Style
Michelle Di Palma. (Thu, 02/25/2021 - 14:22). COVID-19 vaccines. ASU - Ask A Biologist. Retrieved from https://askabiologist.asu.edu/covid-vaccine
Chicago Manual of Style
Michelle Di Palma. "COVID-19 vaccines". ASU - Ask A Biologist. 25 Feb 2021. https://askabiologist.asu.edu/covid-vaccine
MLA 2017 Style
Michelle Di Palma. "COVID-19 vaccines". ASU - Ask A Biologist. 25 Feb 2021. ASU - Ask A Biologist, Web. https://askabiologist.asu.edu/covid-vaccine

During the COVID-19 pandemic, many artists have painted street art as images of hope. Painting by Will Phillips.
Try our COVID simulator to experiment with how different safety measures can affect the spread of the virus that causes COVID-19.
Be Part of
Ask A Biologist
By volunteering, or simply sending us feedback on the site. Scientists, teachers, writers, illustrators, and translators are all important to the program. If you are interested in helping with the website we have a Volunteers page to get the process started.